Fluxgate sensors are precise vector sensors of magnetic fields (can measure both magnitude and direction).
A fluxgate sensor uses a soft magnetic material (with a low coercive force/field and high permeability) as its core material and operates under magnetic saturation state. In saturation, the permeability of the core drops, causing the flux associated with the magnetic field decreases.
Fluxgate Sensor Principle
The term “fluxgate” comes from this “gating” or limiting of the flux at saturation point. The core is magnetically saturated alternatively in opposing directions, normally by means of an excitation coil driven by a sine or square waveform.
Thus, an alternating cycle of magnetic saturation in both direction is formed: magnetized (to the saturation point) → unmagnetized → inversely magnetized (to the saturation point in the opposite direction) → unmagnetized → magnetized (to the saturation point).
This constantly changing field induces a current in the second (sensing) coil. Note that the peak of the excitation current should be big enough to ensure the core to be saturated. In the absence of an external magnetic field, the input and output currents will match.
However, when the core is exposed to an external magnetic field, it will be more easily saturated in alignment with that field direction and less easily saturated in the opposite direction. Hence, the alternating magnetic field, and the induced output current, will be out of phase with the input current.
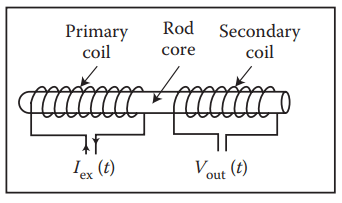
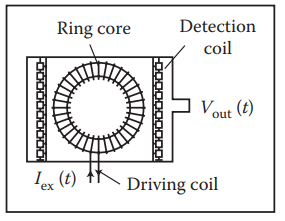
Some fluxgate sensors operate with current sensing in the pickup (secondary) coil, and others with voltage sensing in the pickup (secondary) coil. No matter which mechanism is used, the magnitude of the output signal should be proportional to the strength of the external magnetic field to be measured.
Features of Fluxgate Sensors
For low magnetic field sensing, the fluxgate sensors provide the best trade-off between cost and performance. It can measure both magnitude and direction of a DC (static) or low-frequency AC magnetic field in the range of 0.1 nT – 0.1 mT with the achievable resolution of 0.0001 μT.
Many fluxgate sensors have a bandwidth of a few hertz to kilohertz frequencies.
Fluxgates can operate over a wide temperature range. Typical temperature stabilities drift less than 0.1 nT ⋅ °C−1 with a temperature coefficient around 30 ppm ⋅ °C−1. Some fluxgates can be compensated to 1 ppm ⋅ °C−1.
In terms of dynamic range and resolution, fluxgate sensors perform better than the Hall effect sensors and are preferable to superconducting quantum interference devices (SQUIDs) because of their lower cost and size. If the fluxgate operates in feedback mode, the linearity error may be as low as 10−5.
Fluxgate sensors, using soft magnetic materials as their core materials (with low coercitive field Hc), have the following characteristics:
- Low losses at the excitation frequency (usually in the tens of kilohertz range)
- A low saturation induction value (implies a low power consumption)
- A minimal magnetostriction effect
- Low magnetic noise due to easy reversibility of the magnetization
These important features have made fluxgate sensors ideal for a variety of applications.
Design and Applications of Fluxgate Sensors
Fluxgate sensor designs fall broadly into two styles, those employing rod cores and those using ring cores.
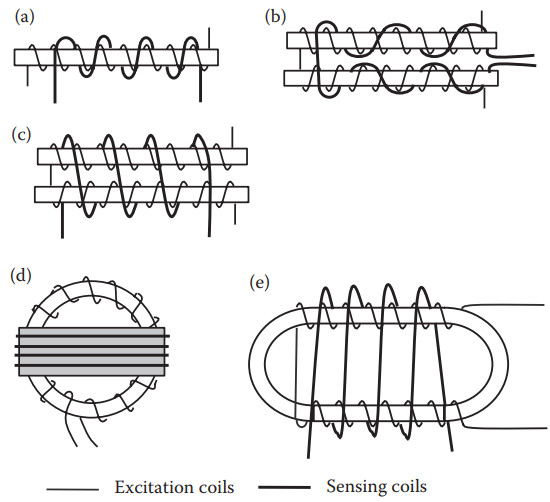
The rod cores can be further divided into a single-rod (Figure 3a) or a twin-core design.
Twin cores are further split into two categories: the Forster (Figure 3b) and Vacquier (Figure 3c) based.
A ring core can have either a circular (Figure 3d) or race-track (Figure 3e) shape.
In a single rod-shaped core, the sense coil will not only pick up the signal voltage, but also the excitation drive, which due to its high level can be troublesome to remove electronically. A common solution for this is to use two parallel cores with the excitation winding reversed from one to the other. Thus, the sensing coil picks up only the signal voltage, while the induced the excitation voltage is cancelled by the phase reversal.
The only difference between a Forster and a Vacquier is their sensing coil configuration as shown in Figure 3b and c.
In the Forster design, the sensing coils indicated by the darker ones in Figure 3b are wound such that the excitation affection is cancelled out, whilst the useful voltage (output) signals are combined. It is also possible to build a fluxgate based on the Forster using a common set of coils for excitation and sensing.
The core material for the rod in the Forster design is traditionally high mu-metal wire (e.g., 77% nickel, 16% iron, 5% copper, and 2% chromium or molybdenum). The rod is typically 20 mm in length but can vary from 15 to 75 mm.
The long-term stability of this type of sensor is better than 3 nT drift per year [31]. The sensor’s sensitivity direction is along the core’s longitudinal direction. This type is often used in geophysical observatory. The Vacquier configuration is often used in satellite instruments.
Unfortunately, most of the amorphous wires exhibit large noise when a core has a long dimension. For example, Vacquier-type sensors made of stress-annealed Vitrovac 6025 amorphous tape exhibited a minimum noise of 11 pT and 46 pT RMS (60 MHz – 10 Hz) for 65 and 35 mm long sensors, respectively. It has been shown that the cores and sensing coils should be 10% shorter than the excitation coils to reduce noise caused by unsaturated ends.
A ring core can be considered as the connection of the tops and the bottoms of the twin rods to form a continuous magnetic loop. It normally uses a toroidal excitation winding spread evenly around the core (Figure 3d), with a sensing coil wound around the outside and orthogonal to the core plane, similar to the Vacquier sensing winding.
The axis of the sensing or feedback winding defines the sensitive direction of the sensor, since the core itself is isotropic. It is common for a single axis sensor to have its sensing winding completely cover the ring core in order to achieve better performance.
For two-dimensional (XY) sensing, two sets of sense coils are put over the toroid at 90° angle to one another to create two sensitive axes. These coils, however, are usually short to avoid cross coupling.
In a tri-axis system, the sensor can be built in a similar way with two rings. The ring can be a simple ferrite core or tape wound core using permalloy or amorphous metal tapes of soft magnetic materials.
Ring core fluxgates are broadly used in modern satellite missions for mapping the magnetosphere and deep space explorations. The ring-core geometry has advantages of creating lower noise and using less power consumption than rod core sensors.
Ring-core sensors also demonstrate less stability offset than other shape cores for the same length. However, ringcore sensors have low sensitivity due to large demagnetization. Race-track sensors, however, have higher sensitivity than other types due to the lower demagnetization factor.
Race-track shape cores have a problem of producing large spurious signals that cannot be easily balanced as the ring cores do (by rotation to minimize the spurious components). One solution is to change the track width along the core length to adjust for unbalance caused by core or excitation winding nonhomogeneity.
A superior design is to add a feedback coil (or double up the sensing coil for this purpose) to feedback a magnetic field in opposition to the sensed field such that the two fields cancel one another. In this mode of operation, the fluxgate is used as a null detector, and the current in the feedback coil is proportional to the sensed field. This technique improves linearity of measurement, allows a much greater dynamic range to be measured, and is used by the majority of modern devices.
In recent years, PCB (printed circuit board) planar fluxgates or micro-fluxgates have increasing applications due to their low cost and easy fabrication.
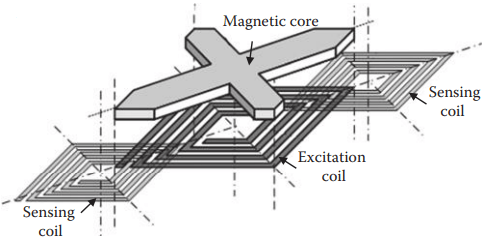
Figure 4 shows a structure for a differential single-axis planar fluxgate magnetic sensor. The ferromagnetic core is placed over the diagonal of the excitation coil. An AC current in the excitation coil saturates each half of the core periodically in opposite directions. If no external magnetic field is present, the output voltage of two sensing coils is zero.
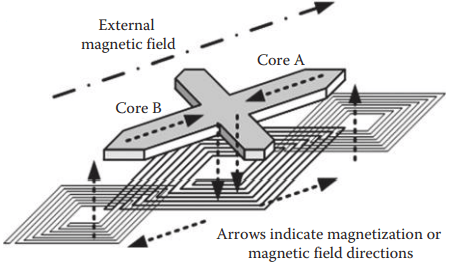
When an external magnetic field is present and parallel to the core, the magnetization in one half of the core is in the same direction as the external magnetic field, while the magnetization of the other half of the core is in the opposite direction (see Figure 5). Thus, the voltage induced in the two sensing coils is not equal and the differential output voltage increases its value.
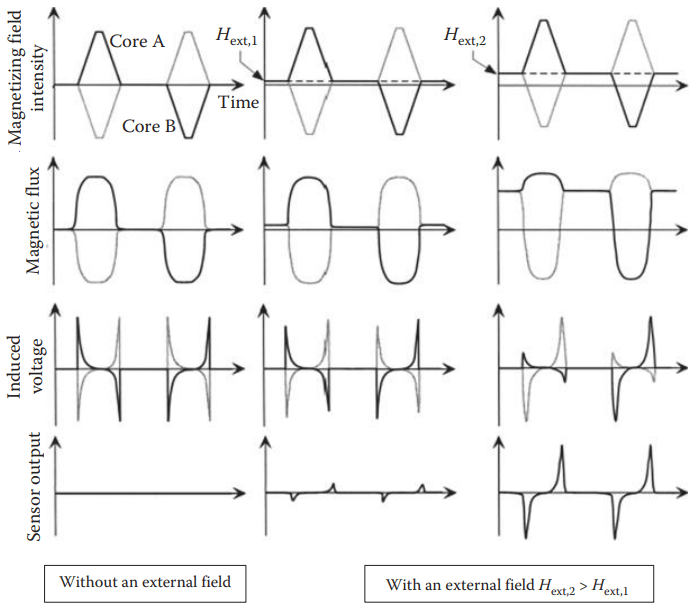
Figure 6 shows the magnetization process and the sensor’s outputs with or without an external magnetic field. Many planar fluxgate sensors do not require electroplating or sputtering processes for ferromagnetic material deposition because very thin amorphous metals are commercially available (e.g., 25 μm for Vitrovac 6025X; 20 μm for Vitrovac 6025Z; and 16 μm for Metglas 2714A) with very good magnetic characteristics.
Fluxgate sensors are commonly used for high-resolution measurements at low frequencies in applications where the sensor must operate at room temperature. Simple fluxgate sensors can detect metal objects and read magnetic marks/ labels. Fluxgate compasses are used in aircraft, land vehicle, and submersible navigation systems.
The fluxgate principle is also used in electrical current sensors and current comparators. The most common use of fluxgate sensors is in static magnetic field measurement.
Fluxgate sensors can also measure alternating fields up to several kilohertz, depending on excitation frequency, the core, sensing and pickup coil designs, and circuits used. Followings are some application examples.
DC Magnetometer
In a DC magnetometer, there are separate pickup and feedback coils. The core, in most cases a ring core, is periodically saturated in both directions by excitation current in the primary coil. When an external field (to be measured) appears, it alters the harmony and voltage (or current if it is a short-circuited mode) induced in the pickup (secondary) coil, which are measured by a phase detector and fed back as a current into a compensation coil. The integrator in the feedback loop ensures enough DC gain and makes the loop to respond to only low-frequency (DC) magnetic field.
PCB Planar Fluxgate for Electronic Compass
Figure 7 shows a single-axis planar fluxgate magnetic sensor using an excitation (primary) coil (30 turns, 30 μm thick, 25.12 mm × 31.3 mm) and two sensing coils (30 turns, 17 μm thick, 31.3 mm × 31.3 mm) configured in differential format with metal lines’ pitch of 400 μm.
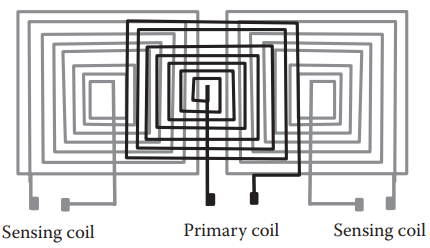
The excitation and sensing coils are placed on two different metal layers in a multilayer PCB structure. The ferromagnetic sheet core is a special amorphous alloy (Vitrovac 6025, μr ≅ 105, magnetic saturation at 0.55 T).
Thanks for reading about “fluxgate sensor principle”.
This is Park who work in the field of NDT using magnetoc sensor. If you send me this article, it will be great help to me.
Sincerely, Park
[email protected]
This article is taken from a book. I will send you that book in some days.